Scientists Across Globe Build Upon Previous Breakthrough
Researchers from institutions across the world proved with real materials what theoretical physicists have said to be true: isotope-enriched cubic boron nitride has ultrahigh thermal conductivity.
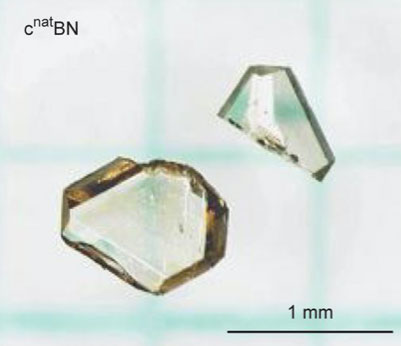
The researchers, including University of Houston M.D. Anderson Chair Zhifeng Ren, recently published their findings in Science. Ren is the director of the Texas Center for Superconductivity at UH (TcSUH) and professor of physics at UH’s College of Natural Sciences and Mathematics.
The group found that isotopically pure cubic boron nitride has 75% of the thermal conductivity of diamond.
The breakthrough could ultimately lead to better-performing cell phones and high-powered devices.
Nearly as Conductive as Diamond
Diamond remains the material with the highest thermal conductivity, but it is expensive. Both isotope-enriched cubic boron nitride and boron arsenide come close to its thermal conductivity.
Thermal conductivity is measured in the unit of Wm-1K-1, watts per meter-kelvin. It is the amount of heat that can pass through a material that is one meter long when the temperature difference from one side to the other is one-degree Kelvin.
The cubic boron nitride crystals that researchers at Japan’s National Institute for Materials Science grew have a thermal conductivity of 1600 watts per meter-kelvin at room temperature in samples with enriched boron-10 or boron-11, the isotopes of boron. The thermal conductivity of diamond is 2200 watts per meter-kelvin. These two boron isotopes allow the crystal vibrations that carry heat to move more efficiently through the material.
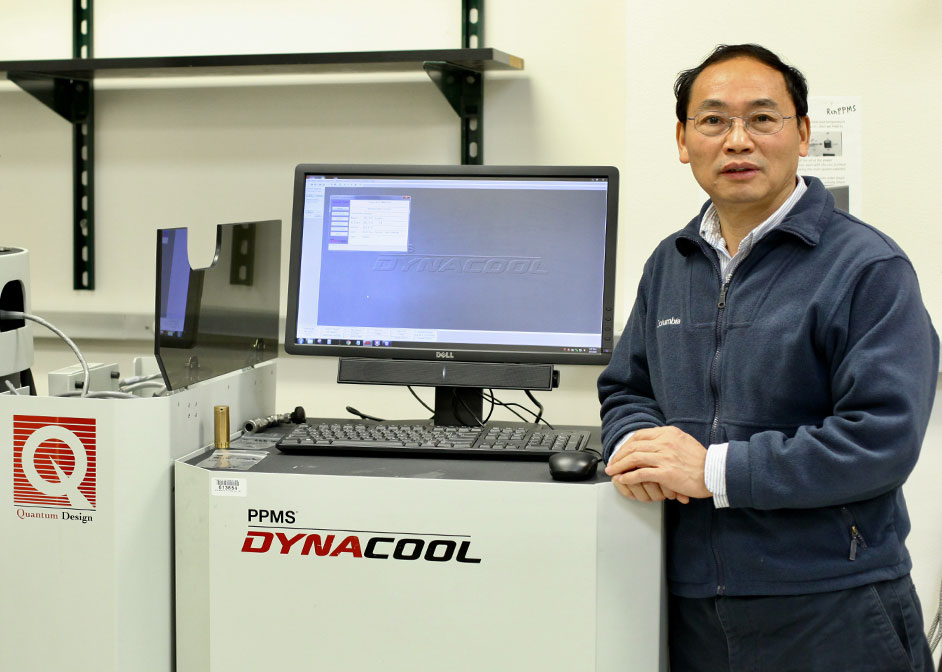
The discovery of the ultrahigh thermal conductivity of cubic boron nitride comes after a breakthrough Ren and his research group at UH made in 2018 on boron arsenide and published in Science. The group created a boron arsenide crystal with thermal conductivity far higher than other semiconductors and metals in use, like silicon, silicon carbide, copper and silver.
Boron Arsenide’s Advantage
Ren and researchers created the boron arsenide crystals using chemical vapor transport. Previous reported efforts to synthesize boron-arsenide yielded crystals that were less than 500 micrometers – too small for useful application.
But Ren and researchers reported growing crystals larger than 4 millimeters by 2 millimeters by 1 millimeter.
Potential Use in High-Powered Computers and Electronics
Both cubic boron nitride and boron arsenide could be used in many devices such as high-powered computers and high-powered electronics that generate large amounts of heat.
“Any time you have a lot of heat, you want to get rid of the heat,” said Ren. “You need a material with high thermal conductivity to get the heat out.”
If heat is not released, Ren adds, the computing power decreases automatically.
Continuously Improving
Ren wants to find an easier way to create materials like boron arsenide.
“When it’s easier to make, obviously the price is also low,” he said. “And when the price is low, you can use it for large-scale applications.”
Ren and the research team have been working on boron arsenide since 2013 and have been continuously improving the quality of the samples ever since.
“Publishing a paper in Science is always exciting because it tells the world that this is important,” said Ren. “It’s always the goal to publish your work in prestigious journals with many readers so that you can create impact.”
With 1 ½ years of funding remaining from the Office of Naval Research, Ren will continue to work on improving the crystal quality of boron arsenide.
Additional researchers involved with this publication include Haoran Sun, Geethal Amila Gamage Udalamatta Gamage and Fei Tian of UH; Ke Chen, Bai Song, Zhiwei Ding, Qichen Song, Aaron Schmidt and Gang Chen of the Massachusetts Institute of Technology; Navaneetha Ravichandran and David Broido of Boston College; Qiye Zheng, Akash Rai, David Cahill of the University of Illinois at Urbana-Champaign; Xi Chen, Hwijong Lee and Li Shi of the University of Texas at Austin; Sheng Li, Hanlin Wu, Pawan Koirala and Bing Lv of the University of Texas at Dallas; and Kenji Watanabe and Takashi Taniguchi of the National Institute for Materials Science in Japan.
- Rebeca Trejo, College of Natural Sciences and Mathematics